From Alchemical Recipes to Modern Chemistry
(2003-10-08)
Black Powder / Blackpowder / Gunpowder
What is the composition of black powder ?
The French call it either poudre à canon (gunpowder)
or poudre noire (blackpowder).
The loose powder was called serpentine.
The name black powder is of relatively recent origin,
as it appeared only after other explosives were devised which lacked the
black luster of free carbon.
Obviously, the stuff wasn't called gunpowder
before the gun was invented, around 1313.
The invention of the gun is often credited to brother
Berthold Schwarz (Schwartz),
a Franciscan friar from Freiburg
with a bogus last name ("Black" in German) indicating
his interest in alchemy, the black art;
the real name of "Black Bert" was most probably Constantine Anelzin.
He "invented" gunpowder only in the sense that he found a new use for old serpentine
and thus made the new name meaningful.
Black powder was the first explosive ever devised,
and it remained the only one for centuries.
It is composed of the following three solid ingredients:
- Saltpeter:
KNO3 niter
(or, more rarely, NaNO3 Chilean nitrate).
- Sulphur: S.
["sulfur" and "sulphur" are equally acceptable spellings]
- Carbon: C.
Often in the impure form of charcoal from wood (willow).
However, simply mixing the ingredients produces only inferior meal powder...
To obtain what's now considered proper black powder,
the ingedients must be "incorporated" in a damp state.
This allows the application of great pressure to form a dense cake,
ultimately broken down into dry grains.
This process is called corning,
and it was first introduced in France in 1429.
Early forms of blackpowder may have existed in China around
AD 700, using crude recipes calling for equal weights of the three components...
Such mixtures would only burn violently without exploding...
Also, explosion cannot occur if raw saltpeter is used,
and the refining of saltpeter is not mentioned before 1240 in a book on
military
technology by the Syrian scholar Hassan Al-Rammah, entitled
al-furusiyya wa al-manasib al-harbiyya.
The first Chinese author to describe an explosive formula was apparently
Huo Lung Ching, in 1412.
In a six-page tract entitled Liber Ignium ("Book of Fires"),
Marcus Graecus [an otherwise unknown author, possibly a fictitious one]
describes 35 incendiary recipes,
including a formula which was once standard for English blackpowder:
[...] 1 lb of native sulfur,
2 lb of linden or willow charcoal, 6 lb of saltpeter,
which three things are very finely powdered on a marble slab.
The latin version of this pamphlet did not appear before 1280 or 1300 and may
have originated around that time, although the claim has been made that
it was an expanded translation by Spaniards of a more ancient Arabic text
(dated AD 848)
and/or a Greek version that did not include the last four formulas...
Roger Bacon (c.1214-1292) investigated black powder before 1249,
when he devised the recipe he communicated in 1268:
40% more saltpeter than
either sulphur or carbon (7:5:5 formula by weight).
However, the first unmistakable blackpowder explosive composition
is the "German formula" (4:1:1) proposed by Albertus Magnus (c.1200-1280).
The English standard formula around 1350 called for less sulphur and more charcoal
(6:1:2).
The most commonly quoted modern gunpowder composition seems to date
from around 1800 and calls for 75% saltpeter (niter) oxidizer, with
10% sulfur (S) and 15% charcoal (C) fuel:
Some Historical Formulae for Black Powder (by weight)
Date | Who / What / Where |
KNO3 | Sulphur | Charcoal |
---|
c. 700 | Chinese alchemists (?) | 1 | 1 | 1 |
1249 | Roger Bacon | 7 | 5 | 5 |
1275 | Albertus Magnus ("German") | 4 | 1 | 1 |
c.1300 | "English" (Marcus Graecus?) | 6 | 1 | 2 |
| Swiss "Bernese Powder" | 76 | 10 | 14 |
1781 | Britain | 75 | 10 | 15 |
1794 | France | 76 | 9 | 15 |
1800 | Prussia | 75 | 11.5 | 13.5 |
Stoichiometry (see below) | 74.8 | 11.9 | 13.3 |
The stoichiometry of the following
oversimplified
reaction would correspond to about
74.8% niter, 11.9% sulphur and 13.3% carbon (roughly 101:16:18):
2 KNO3
+ 3 C
+ S
®
K2S
+ 3 CO2
+ N2
+ 572 kJ
(505.8 cal/g)
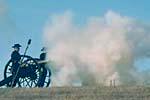
The potassium sulphide
solid residue forms a thick white smoke,
capable of obscuring entire battlefields.
Newer propellants leave little or no such residue when properly exploded.
They are thus collectively known as smokeless powders.
The simplest idea
for a smokeless dark powder is called ammonpulver (AP) and involves
ammonium nitrate (AN) with 10% to 20% charcoal,
although the stoichiometry of the following reactions translates into only
7% to 13% carbon, by weight:
2 NH4NO3
+ C
®
CO2
+ 4 H2O
+ 2 N2
+ 629.6 kJ
(874.4 cal/g)
NH4NO3
+ C
®
CO
+ 2 H2O
+ N2
+ 228.6 kJ
(593.5 cal/g)
Other smokeless powders of historical interest
include the following propellants:
- Guncotton,
or nitrocellulose (also known as pyropowder, pyrocellulose,
trinitrocellulose and cellulose nitrate) invented in 1845
by the Swiss chemist Christian Schönbein (1799-1869).
- Poudre B
(flakes of nitrocellulose gelatinized with ether and alcohol)
invented in 1884 by Paul Vieille (1854-1934)
for the 1886 Lebel rifle.
- Ballistite
(nitrocellulose and nitroglycerin, with diphenylamine stabilizer)
invented by the Swedish industrialist Alfred Nobel (1833-1896) in 1887.
- Cordite N
(nitroguanidine,
nitrocellulose, and nitroglycerin)
invented by Sir Frederick Augustus Abel and Sir James Dewar in 1889.
Sulfurless powder (12.93% carbon) would yield 772.6 cal/g, with 60% smoke:
4 KNO3
+ 5 C
®
2 K2CO3
+ 3 CO2
+ 2 N2
+ 1501.4 kJ
It takes 92.9 g of this mix to release a mole of gas,
whereas only 67.6 g of black powder would suffice
(as sulfur prevents the wasteful production of carbonate).
(2003-11-14)
Simple Predictions of Chemical Outcomes
How do we tell what a given initial composition will produce?
This may be tough, since the result of a chemical reaction is
always an equilibrium containing everything that could be produced
(possibly only in minute quantities).
However, for reactions involving chemical explosives, a decent
rule
of thumb is to use the following hierarchy of
fictitious reactions and consider that
each occurs only when the previous ones have been completed
to the fullest possible extent:
Metal + Oxygen |
® |
Oxide |
C + O | ® | CO |
2H + O | ® | H2O |
CO +
O | ® | CO2 |
Oxide + CO2 | ® | Carbonate |
N, O, or H | ® |
½N2, ½O2, or ½H2 |
C | ® | C (black smoke) |
This is only a rough approximation of chemical reality
(useful, but not foolproof).
(2003-10-09)
Enthalpy of Formation
How do we compute the energy balance of a chemical reaction?
The enthalpy
of formation (DH) of a chemical compound is
roughly
the energy required to make it from its constituents
[in their standard forms, as gases, liquids, or crystals].
Once tabulated,
this data can be used to work out the energy balance
in a reaction involving such compounds.
The so-called bond energy is a misguided poor rule-of-thumb
which is unfortunatly still taught ar the introductory level.
In the few cases where it would be applicable (diatomic molecules) it's almost always
incompatible with the standard enthalpy of formation, which refers to formation
from realistic molecules rather than fictitious isolated atoms.
DH f < 0
for stable compounds (exothermic formation).
Substance |
DH f (kJ/mol) |
---|
potassium carbonate (s) | K2CO3 |
-1150.18 |
calcium dihydroxide (aq.) | Ca++, 2 OH - |
-1003 |
calcium dihydroxide | Ca(OH)2 |
-986.09 |
calcium dihydroxide gas | Ca(OH)2 |
-610.76 |
calcium ion | Ca++ |
-543.00 |
potassium nitrate (nitre) | KNO3 |
-494.60 |
sodium nitrate | NaNO3 |
-467.90 |
carbon dioxide | CO2 |
-393.51 |
potassium sulphide | K2S |
-380.70 |
nitroglycerin |
C3H5(NO3)3 |
-371.10 |
ammonium nitrate (AN) | NH4NO3 |
-365.60 |
water | H2O |
-241.826 |
hydroxide ion | OH - |
-230.015 |
carbon monoxide | CO |
-110.53 |
nitroguanidine | H2NC(NH)NHNO2 |
-91.63 |
calcium carbide | CaC2 |
-59.80 |
trinitrotoluene (TNT) | C7H5N3O6 |
-54.39 |
black phosphorus | P |
-39.30 |
red phosphorus | P |
-17.60 |
white phosphorus (toxic) | P |
0.00 |
phosphorus gas | P4 |
+58.90 |
phosphorus gas | P2 |
+144.00 |
acetylene | C2H2 |
+226.73 |
phosphorus gas | P |
+316.50 |
For example, the energy released in the combustion of CO is
the difference between the enthalpies of
formation tabulated above for CO and CO2 :
CO + ½ O2
® CO2
+ 282.98 kJ
A positive enthalpy of formation indicates an unstable compound, like acetylene,
which would release energy by reverting back to its elemental components.
However, a negative enthalpy of formation is no practical guarantee of stability.
Like liquid
nitroglycerin,
some chemicals do detonate into more stable ones:
4 C3H5(NO3)3
®
12 CO2 + 6 N2 + 10 H2O + O2 + 5656 kJ
(1488 cal/g)
(2003-10-10)
Ink Formulas What is the composition of traditional inks ?
Natural Ink
Sepia is the most lasting of natural inks, but it's not lightfast.
It is a dark brown liquid
consisting of concentrated melanin,
secreted by Mediterranean cuttlefish and other cephalopods
(it's stored in ink sacs and ejected to confuse attackers).
India Ink (Chinese Ink)
As early as 2500 BC, writing inks were carbon inks
consisting of fine grains of carbon black [from soot] suspended in a liquid.
The Latin name for this was
atramentum librarium and it's now called
India ink or Chinese ink.
On the famous Dead Sea Scrolls of Qumran
(from the third century BC to AD 68),
a red version of this ink is found which uses
cinnabar (HgS) instead of carbon.
The idea is simple:
When the liquid dries out, the solid pigment (C or HgS) remains which
leaves a permanent trace.
Such inks are best used on semi-absorbent stuff, like paper or papyrus (not parchment).
The problem was to keep the grains in suspension long enough to apply the ink.
In plain water, fine grains of carbon black would aggregate under the action of
Van der Waals forces and form flakes
large enough to fall quickly to the bottom of the container.
This flocculation process can be prevented with an hydrophilic additive
which minimizes Van der Waals interactions between the grains
by coating them (as was properly explained only in the 1980s).
Early ink recipes may thus have called for various plant juices
instead of plain water.
It turns out that gum arabic acts this way to stabilize
India ink into a colloidal suspension for days or weeks...
This wonderful invention is at least 4500 years old.
Traditional Chinese ink is not bottled.
Instead, ink is produced as needed
by grinding an inkstick on an inkstone after adding a little
water (the inkstone also acts as an inkwell).
Chinese ink-sticks consist of a pigment (usually soot from pine,
oil or lacquer) and a soluble resin which holds the dry stick together
and plays a critical part in the colloidal ink suspension produced by wet grinding.
Iron-Gall Ink, Indelible Ink, Encaustum
In the first century AD, Pliny the Elder described a basic chemical demonstration
of the principle behind what would become the primary ink of the Middle Ages:
Papyrus soaked in tannin turns black upon contact with a solution of iron salt.
This was not used for actual ink at the time of Pliny,
but "gallarum gummeosque commixtio" is already mentioned as
an established writing ink around AD 420,
in the
encyclopedia of the 7 liberal arts by Martianus Capella.
However, the latest analyses have disproved dubious reports that this type of ink might
have already been used on the famous Dead Sea Scrolls of Qumran (before AD 68).
Because of the secondary reaction discussed below, which makes it indelible,
iron ink was once known as encaustum
(Latin for "burned in", from the Greek enkauston, meaning
painted in encaustic and fixed with heat).
This is the origin of the English word "ink" itself,
and of its counterparts in a number of other languages:
encre (French),
inchiostro (Italian),
inkt (Dutch),
inkoust (Czech)...
Indelible iron-gall ink is considered the most important ink in the development
of Western civilization, up until the 20th century.
The best iron-gall inks were far superior to most modern inks,
but the corrosiveness of some compositions (discussed below)
regretfully led to the abandonment of all iron-gall inks in favor of
more sophisticated recipes with lesser chemical aggressivity.
Iron-gall ink normally includes what is effectively a
"Chinese ink" component, which provides both body (from gum arabic)
and some initial coloring upon application of the ink.
Otherwise, the main pigmentation of iron-gall ink comes paradoxically from
water-soluble ferrous chemicals with little color of their own:
When the ink dries in air, an oxidation occurs which turns these
ferrous salts into insoluble ferric dark pigments.
In addition, iron-gall ink may react with parchment collagen or paper
cellulose, in a totally indelible way.
Some poorly balanced iron-gall inks have even been observed to
burn holes through paper.
It has been shown
that an excess of ferrous salt in iron-gall ink
leaves permanent traces of active soluble salts
(not properly oxidized into inert pigments) which will catalyze
the slow decomposition of cellulose, especially when acidity is present.
This corrosion is reduced with a proper balance in the composition of the ink.
To prevent deterioration of historical iron-gall ink documents,
the Netherlands Institute of Cultural Heritage (ICN) has introduced an interesting
treatment,
which was first used on a large scale by the conservators of the
Nationaal Archief of the Netherlands:
First, a saturated solution is applied which contains a calcium salt and its acid, namely:
The salt is soluble up to twice the molar concentration of the acid.
This is an oxidation inhibitor which binds the metal ions.
Then, acidity is neutralized with calcium bicarbonate,
which creates an alkaline buffer and also leaves a phytate precipitate in the fibers,
for continued oxidation protection.
Iron-nutgall ink, tannin Ink, gallotannate ink, vitriolic ink.
Modern Inks
Key Ink Ingredients:
- Gum arabic:
Exuded by acacia
(acacia
senegal) and other African trees,
it is a very common thickener and colloidal stabilizer.
Some candies are made from up to 45% gum arabic.
Also called acacia.
[info]
CAS 9000-01-5: Gum acacia; gum arabic; acacia gum; Indian gum.
- Ferrous sulfate:
Also known as kankatum, green vitriol or copperas.
(FeSO4, 7 H2O) iron sulphate
in hydrated crystal form (278.01 g/mol).
- Tannin: Tannic
(or gallotannic) acid,
extracted by water-saturated ether from crushed gallnuts
( galls, nutgalls, or gall apples ).
It is an anhydrid of gallic acid (see next):
COOH.C6H2(OH)2O.COC6H2(OH)3
- Gallic acid:
Produced (with glucose)
by the hydrolysis of tannin in acid.
Used in calotype
photography.
C6(COOH)H(OH)3H (170.12 g/mol)

Pigments:
- Carbon Black :
Lampblack, from soot. C (12.01 g/mol)
- Manganese Black :
Manganese dioxide. MnO2 (86.937 g/mol)
- Cinnabar :
Called vermillion, or Chinese red. HgS (232.66 g/mol)
- Red Ochre :
Hematite. Ferric oxide. Fe2O3 (159.69 g/mol)
- Sepia :
Natural sepiomelanin from sepia officinalis.
[ 1
| 2
]
- Viridian :
Chromium oxide dihydrate.
Cr2O3 . 2 H2O (Guignet, 1859)
- Green Malachite :
Basic cupric carbonate.
CuCO3-Cu(OH)2
- Egyptian blue :
Synthetic cuprorivaite.
CaCuSi4O10
3100 BC
- Indigo :
"Indian Blue".
CAS 482-89-3
C16H10N2O2
1580 BC
- Maya Blue :
Palygorskite clay and indigo complex.
[ 1 |
2 |
3 |
4 ]
- Lapis Lazuli :
Lazurite (sodium aluminum silicate)
not "lazulite".
[supplier]
(Na,Ca) 8 (AlSiO4 )6
(S, SO4 , Cl 2 )
especially:
Na 8 (AlSiO4 )6 S.
- Prussian Blue :
Ferric ferrocyanide.
Ferric hexacyanoferrate.
Fe4 [Fe (CN)6 ] 3
A chelating agent insoluble in water (Diesbach, 1704).
Iron Gall Ink
|
How to Make Iron Gall Ink
|
Ink Corrosion
|
Old Ink
|
Period Inks
Forty Centuries of Ink
|
Ink Recipes
|
Gallotannin
|
Pigment Chemistry
|
Rare Oil Colors
(2003-10-11)
Redox Reactions
An oxidizer gains the electrons which a reductant loses.
Redox reactions
are best described as transfers of electrons between chemical species:
An oxidizer (oxidant, or oxidizing agent) is "reduced" by gaining electrons;
a reducer (reductant, or reducing agent) is "oxidized" by losing them.
Some Redox Half-Reactions | Potential
(25°C, 1 atm) |
Fluorine | ½ F2
+ e- |
® |
F- |
(+2.866 V) |
Gold (aurous) | Au+
+ e- |
® |
Au |
(+1.692 V) |
Permanganate | MnO4-
+ 4 H +
+ 3 e- |
® |
MnO2 + 2 H2O |
(+1.679 V) |
Permanganate | MnO4-
+ 8 H +
+ 5 e- |
® |
Mn++ + 4 H2O |
(+1.507 V) |
Gold (auric) | Au+++
+ 3 e- |
® |
Au |
(+1.498 V) |
Nitrate | NO3-
+ 2 H +
+ e- |
® |
NO2
+ H2O |
|
Chlorine | ½ Cl2
+ e- |
® |
Cl- |
(+1.35827 V) |
Copper (cupric) |
Cu++
+ 2 e- |
® |
Cu |
(+0.3419 V) |
Acid |
H +
+ e- |
® |
½ H2 |
( 0 V ) |
Methanoate (or formate) |
CO2
+ H +
+ 2 e- |
® |
COOH - |
|
Ethanedioate (or oxalate) |
2 CO2
+ 2 e- |
® |
C2O4- - |
|
Zinc |
Zn++
+ 2 e- |
® |
Zn |
(-0.7618 V) |
Lithium |
Li+
+ e- |
® |
Li |
(-3.0401 V) |
Each of the above half-reactions is written as the reduction
of an oxidizer, but the reverse direction (the oxidation of the reducer on the
right-hand side) is more common for the reactions with a low
redox potential (listed in volts V):
In a complete redox reaction,
a reduction occurs as written above
only if a balancing oxidation
with a lower redox potential occurs in the reverse direction.
For example, the nitrate ion has a higher potential than the cupric ion and
nitric acid may thus oxidize copper metal.
(The opposite relation holds between hydrogen and cupric ions,
so an ordinary acid can't oxidize copper.)
2 NO3-
+ 4 H +
+ Cu
®
2 NO2
+ 2 H2O
+ Cu++
In a balanced redox reaction, the difference DE
between the potentials of both half-reactions is simply
the change in free enthalpy DG
(G = H-TS) per unit of electric charge transferred.
If n moles of electrons are involved, this translates into
n moles of electronvolts in DG
for each volt in DE. Therefore:
DG
= -n F DE
= -n DE
(96485 J/V)
= -n DE (23.06 kcal/V)
A joule per volt (J/V) is a coulomb (C) and the above
bracketed constant is the Faraday constant
( F, the charge of a mole of electrons)
in two different units.
Only the DE (or DG)
of an actual redox reaction has a physical meaning,
while all the half-reactions are convenient fictions
whose redox potentials are defined within an additive constant,
which is conventionally set to 0 V for hydrogen.
[Another convention is used for the related "Oxydo-Reduction Potential" (ORP)
measured directly for aqueous solutions, which lets 1 V be the ORP of chlorine.]
The standard redox potential
(DEo )
tabulated for a normal pressure of 1 atm (101325 Pa)
at 25°C (77°F) is understood for unit
(1M) concentrations of
both reactants and products, otherwise the so-called
Nernst equation
is used:
DG =
DGo +
|
RT |
ln ( |
[products] |
) |
 |
[reactants] |
|
DE =
DEo -
|
RT |
ln ( |
[products] |
) |
 |
 |
nF |
[reactants] |
Therefore, even if the comparison of standard redox potentials seems to
imply that a reaction does not occur, what actually evolves is an equilibrium
where the concentration of "products" is small, or even utterly negligible...
(2003-11-01)
Gold Chemistry
Aqua regia, the "Royal Water" which dissolves gold and platinum.
Like silver, gold is impervious to ordinary acids like hydrochloric acid
( HCl, formerly called muriatic acid,
"marine acid" or "spirit of salt").

Unlike silver, gold cannot be oxidized by nitric acid
(aqua fortis)...
However, early alchemists did discover that a mixture of nitric and
hydrochloric acids was able to dissolve gold, the so-called royal metal.
They dubbed the potent mixture "Royal Water", aqua regis or
aqua regia.
Aqua regia is already mentioned in the world's
first
encyclopedia,
published in AD 77 by Pliny the Elder
(Gaius Plinius Secundus, AD 23-79).
Aqua regia is a mixture of at least 3 moles of
hydrochloric acid per mole of nitric acid
(it's better to have too much hydrochloric acid than too little).
It's used concentrated and hot for best efficiency.
Aqua regia is also called chloroazotic, chloronitric,
nitromuriatic, or nitrohydrochloric acid
("eau régale" in French).
Nitrosyl chloride
and chlorine fumes are evolved upon mixing:
HNO3 + 3 HCl
®
NOCl + Cl2 + 2 H2O
The chemical equilibrium for the oxidation of gold by the nitrate ions in nitric
acid would only result in a minute concentration of auric cations
[Au+++], but in aqua regia
the concentration of auric ions is constantly depleted because
auric cations combine quickly with chlorine anions to form complex
chloroaurate ions:
Au+++ + 4 Cl -
®
AuCl4-
The speed of the overall reaction is limited by the [Au+++ ]
concentration from the redox equilibria.
As this improves with temperature, aqua regia may be used
at 100°C or more (in a bath of boiling salty water).
Gold may form compounds in two oxidation states +1 (aurous)
and +3 (auric):
- Byproducts or reactants in the electrolytic refining of gold:
- CAS 10294-29-8:
Aurous chloride / Gold monochloride (AuCl).
- CAS 13453-07-1:
Auric chloride / Gold trichloride (AuCl3).
- CAS 16903-35-8:
Chloroauric acid (HAuCl4).
- CAS 16961-25-4:
--- trihydrated crystals
(HAuCl4, 3 H2O).
Note: The term "gold chloride"
is unfortunately used for any of the above!
- Gold-plating baths: (potassium aurocyanide, potassium gold cyanide).
- CAS 13967-50-5:
Potassium dicyanoaurate K[Au(CN)2].
- Rheumatoid arthritis medicine:
- CAS 15189-51-2:
Sodium aurichloride (NaAuCl4, 2 H2O).
The combination of gold trichloride with the chloride of
another metal is called an aurochloride, aurichloride,
chloraurate or [preferably] chloroaurate.
 Fulminating Gold, the First High Explosive:
Since gold is so difficult to combine with other elements,
all gold compounds are fairly unstable.
Some much more so than others, though:
In 1659, Thomas Willis and Robert Hooke demonstrated
that a powder of gold hydrazide explodes on a mere concussion,
without the need for air or sparks (which were once thought to be required
for any kind of ignition).
Gold hydrazide (also known as aurodiamine) is a water-soluble substance
obtained by letting an ammoniacal solution react with an auric hydroxide precipitate
(itself obtained from a gold solution prepared with aqua regia).
Gold hydrazide has a dirty olive-green color (AuHNNH2 ).
Gold hydrazide is apparently only one of several explosive compounds which have been called
fulminating gold (aurum fulminans).
Around 1603, another kind of fulminating gold ("Goldkalck" or "Gold Calx")
was described as the precipitate of gold by potassium carbonate.
These kinds of "fulminating gold" are distinct from "gold fulminate",
the gold salt of fulminic acid (CNOH), another expensive explosive...
In spite of its price, fulminating gold is said to have been used militarily in 1628.
The discovery of fulminating gold has been attributed to the
alchemist Basil Valentine (Basilius Valentinus)
a legendary benedictine monk who is regarded by some
as the "father of modern chemistry" [see next article].
We're told Basil Valentine was born in 1394,
although his main work (The Twelve Keys of Basil Valentine)
was first published only in 1599.
(2003-12-03)
Forefathers of Modern Chemistry
What alchemist or early chemist is the father of modern chemistry ?
Chemistry is a science with many "fathers".
Here are some popular contenders for the title...
- Pliny the Elder, Gaius Plinius Secundus (AD 23-79).
- Geber, Abu Musa
Jabir Ibn Hayyan
(c.740-803).
  - St.
Albert the Great, Albertus Magnus (1205-1280)
- Roger Bacon (c.1214-1294)
- Basil Valentine (1394-14??)
  - Paracelsus
(1494-1541)
- Sir Francis Bacon (1561-1626)
- Robert Boyle (1627-1691)
  - Antoine
Lavoisier (1743-1794)
- John Dalton (1766-1844)
- Amedeo Avogadro (1776-1856)

- Humphry Davy (1778-1829)
- Jöns Jakob Berzelius (1779-1848)
Arguably, chemistry became a science when
Antoine Lavoisier established that
mass is conserved in any chemical reaction,
about which he stated:
Rien ne se perd, rien ne se crée, tout se transforme.
It's only with the advent of Relativity Theory,
that this fundamental conservation law would be proved to be only a first
approximation, albeit an excellent one:
Unlike what happens in nuclear reactions,
the relative variation of mass involved in chemical reactions is so minute
that it can't be measured directly.
The
Fathers of...
|
Geber
|
Chemists
that Shaped the Science
|